A team of scientists studied the inner workings of a perovskite material to better understand the material’s behavior at the atomic scale. Their work revealed that a liquid-like motion in perovskites may explain how they efficiently produce electric currents.
The scientists explained that when light hits a photovoltaic material, it excites electrons, prompting them to “pop out” of their atoms and move through the material, conducting electricity. One problem is that the excited electrons can recombine with the atoms instead of traveling through the material. This can cut the amount of electricity produced relative to the amount of sunlight that hits the material.
Perovskites do well at preventing this recombination, the scientists said. Their work aimed to uncover what mechanism causes this and how more efficient solar cells can be developed.
Duke University led the effort that included scientists at the U.S. Department of Energy’s Argonne National Laboratory and Oak Ridge National Laboratory.
The team studied one of the simplest perovskites, a compound of cesium, lead and bromine (CsPbBr3). They then used X-ray scattering capabilities at Argonne’s Magnetic Materials group’s beamline.
The team captured the average positions of the atoms in a perovskite crystal at different temperatures. They found that each lead atom and its surrounding cage of bromine atoms formed rigid units that behaved like molecules. In particular, the units oscillated in a liquid-like manner.
One theory to explain how perovskites resist recombination is that these distortions in the lattice, or crystal structure, followed the free electrons as they traversed the material. The electrons might deform the lattice, causing the liquid-like disturbances, which prevented them from falling back into their host atoms. The researchers said this theory may offer new insights into how to design optimal perovskite materials for solar cells.
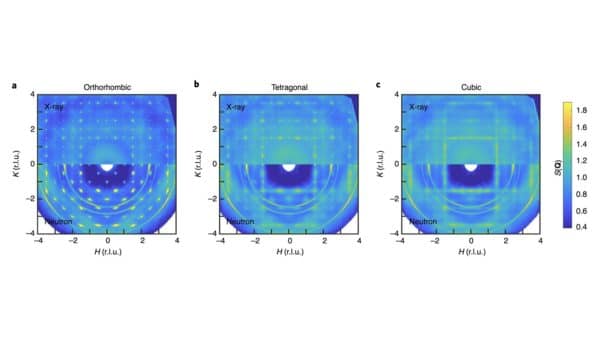
Image: Argonne National Laboratory
The data also indicated that molecules in the material oscillated within two-dimensional planes, with no motion across planes. This two-dimensional nature could also help explain how the perovskite can prevent electron recombination, contributing to the materials’ efficiency.
To investigate the motion of the atoms directly, the team used neutron scattering capabilities at Oak Ridge National Laboratory. Neutron scattering confirmed the pattern seen in the X-ray scattering experiment. It also showed that it took almost no energy for the molecules to oscillate in two dimensions. The researchers said this helps to explain why the excited electrons could deform the lattice so easily.
A paper on the study, “Two-dimensional overdamped fluctuations of the soft perovskite lattice in CsPbBr3,” was published in Nature Materials in March. Computational studies to support the experiment were performed at the National Energy Research Scientific Computing Center at Berkeley National Laboratory. The research was funded by DOE’s Office of Basic Energy Sciences, Materials Science and Engineering division.
This content is protected by copyright and may not be reused. If you want to cooperate with us and would like to reuse some of our content, please contact: editors@pv-magazine.com.
By submitting this form you agree to pv magazine using your data for the purposes of publishing your comment.
Your personal data will only be disclosed or otherwise transmitted to third parties for the purposes of spam filtering or if this is necessary for technical maintenance of the website. Any other transfer to third parties will not take place unless this is justified on the basis of applicable data protection regulations or if pv magazine is legally obliged to do so.
You may revoke this consent at any time with effect for the future, in which case your personal data will be deleted immediately. Otherwise, your data will be deleted if pv magazine has processed your request or the purpose of data storage is fulfilled.
Further information on data privacy can be found in our Data Protection Policy.